Understanding Western Blot: A Comprehensive Guide
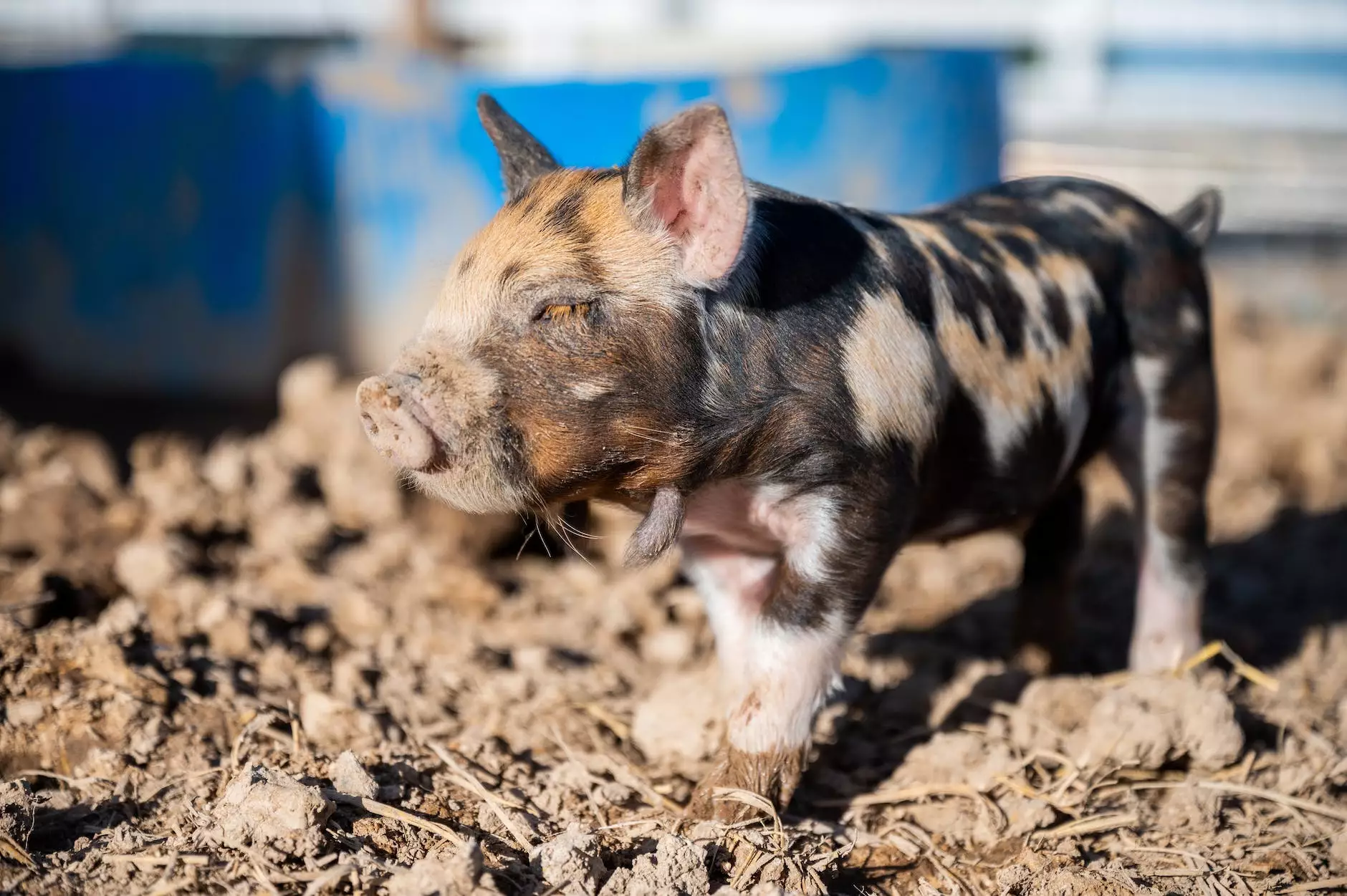
Western blotting is a powerful and sophisticated technique widely utilized in molecular biology, biochemistry, and medical diagnostics. This method is pivotal for detecting specific proteins in a sample, offering invaluable insights into various biological processes, disease mechanisms, and cellular functions. In this article, we will delve into the intricacies of the western blot technique, exploring its applications, methodologies, and the latest advancements shaping this field.
What is Western Blotting?
The Western blot technique is named after the transfer method it employs. It begins with the separation of proteins based on their molecular weight through gel electrophoresis. Following this, the proteins are transferred onto a membrane, typically made of nitrocellulose or PVDF (polyvinylidene fluoride). This transfer is crucial for subsequent detection phases. The method was first established by W. Neal Burnette in 1979 and has since evolved into a fundamental procedure in protein analysis.
The Significance of Western Blotting
Western blotting holds substantial significance in various fields, including:
- Medical Diagnostics: This technique is used for detecting specific proteins associated with diseases, such as HIV, Lyme disease, and various cancers.
- Biomedical Research: Researchers utilize western blotting to study protein expression and post-translational modifications, aiding in the understanding of cellular functions.
- Drug Development: It plays a critical role in the validation of therapeutic targets by providing evidence of protein interactions and expressions.
Understanding the Methodology of Western Blotting
Western blotting involves several critical steps, each essential for ensuring accuracy and reliability. Here’s a comprehensive breakdown of the process:
1. Sample Preparation
Firstly, the biological samples, such as tissue extracts, culture media, or cell lysates, need to be prepared. Proteins are extracted using lysis buffers that may include detergents, salts, and protease inhibitors to ensure protein stability. This step often employs methods like sonication or freeze-thaw cycles to lysate cells effectively.
2. Gel Electrophoresis
The next step involves gel electrophoresis, where the extracted proteins are loaded into a polyacrylamide gel and separated based on their size. When an electric current is applied, smaller proteins migrate faster through the gel than larger ones, creating distinct bands representing different proteins.
3. Protein Transfer
Once the proteins are separated, they must be transferred onto a solid support membrane. This is typically achieved through:
- Wet Transfer: Involving the use of a buffer to facilitate the migration of proteins from the gel to the membrane.
- Semi-Dry Transfer: Allowing for faster protein transfer without the need for soaking in large volumes of buffer.
- Western Transfer: A newer approach that provides higher efficiency and lower background noise.
4. Blocking the Membrane
After the transfer, the membrane is blocked to prevent non-specific binding of antibodies. Common blocking agents include bovine serum albumin (BSA), non-fat dry milk, or commercial blocking buffers. This step is crucial for achieving high specificity in subsequent detection steps.
5. Antibody Incubation
The next phase involves incubating the membrane with primary antibodies that specifically bind to the target protein. After the incubation, the membrane is washed to remove unbound antibodies and then incubated with secondary antibodies conjugated to a detectable marker, such as an enzyme or fluorescent dye.
6. Detection
Finally, the bound antibodies are visualized. Enzymatic substrates can be added for colorimetric detection, while chemiluminescent substrates can be used for enhanced sensitivity with X-ray films or imaging systems. Digital imaging systems also allow for quantification of the results, providing precise data regarding protein expression levels.
Applications of Western Blotting
The applications of the western blot technique span across various disciplines, embodying its versatility and importance:
Medical Diagnostics
Western blotting is one of the confirmatory tests for HIV diagnosis, used to detect specific proteins indicative of the virus. Similarly, it can assist in diagnosing other infectious diseases and autoimmune disorders by identifying specific antigen-antibody interactions.
Cancer Research
In cancer biology, western blotting is employed to investigate alterations in protein expression that may correlate with tumorigenesis or disease progression. By analyzing markers of apoptosis, proliferation, and signaling pathways, researchers can glean insights into therapeutic targets and treatment efficacy.
Neuroscience
In the realm of neuroscience, western blotting is critical for studying neurodegenerative diseases. It enables the detection of proteins such as tau and amyloid-beta associated with Alzheimer's disease, offering potential pathways for developing therapeutic strategies.
Pharmaceutical Development
Pharmaceutical companies leverage western blotting during the drug development pipeline. By monitoring target protein engagement, expression levels, and biological responses to new compounds, it serves as a reliable method in preclinical and clinical trials.
Challenges and Limitations of Western Blotting
Despite its widespread use, several challenges and limitations accompany the western blot technique:
- Specificity Issues: Non-specific bindings can lead to false positives, requiring stringent controls and validation steps.
- Quantitative Limitations: While western blotting can provide qualitative data, quantifying protein levels can be complex and requires appropriate calibration.
- Labor-Intensive: The multi-step nature of the procedure can be time-consuming, necessitating careful optimization of each step for reproducibility.
Recent Innovations in Western Blotting
The field of western blotting is continuously evolving, benefiting from technological advancements that enhance its efficiency and reliability:
1. Automation and High-Throughput Systems
Modern laboratories are increasingly adopting automated systems that streamline the western blotting process, allowing for high-throughput analysis. These innovations reduce hands-on time and improve reproducibility by minimizing operator variability.
2. Enhanced Detection Methods
The advent of advanced imaging techniques, such as mass spectrometry and multicolor fluorescent labeling, has transformed western blot detection. These methods enable multiplexing, allowing for the simultaneous detection of multiple proteins within the same sample.
3. Improved Antibody Selection
With the rise of bioinformatics and antibody validation databases, researchers now have access to a wealth of information about antibody specificity and efficiency. This supports better choices in antibody selection for western blotting, enhancing the overall specificity and sensitivity of the assays.
The Future of Western Blotting
As research continues to advance, the future of western blotting looks promising. Innovations in technology, along with improvements in reproducibility and specificity, position this technique at the forefront of protein analysis. Its adaptability to various biological contexts ensures its relevance for years to come.
Conclusion
In conclusion, western blotting remains an essential tool in molecular biology and diagnostic research. Through its detailed methodology, extensive applications, and continuous innovations, it plays a crucial role in illuminating the complexities of biological processes and diseases. Understanding its mechanisms not only helps researchers and clinicians make informed decisions but also contributes to the broader goal of advancing health and scientific knowledge.